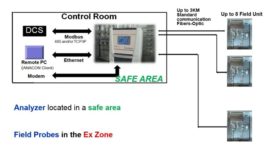
Fluidized Catalytic Crackers are the moneymakers in many refineries as they convert low-cost heavy distillates into high-valued gasoline naphtha. FCC is a complex process where catalytic chemical reactions and fractionating are combined. NIR process analyzers are highly effective in measuring the interlinked physical properties of feedstock, intermediate and final product process streams. Correlation between these, is the keystone to fully optimize the overall process, which definitely increases revenue of the FCC.
Introduction
In the past refineries processed mainly light crude oils, which are the source of relatively large volumes of light distillates, and are excellent to produce gasoline. Gasoline is still a major product refineries deliver to the market. The yield of gasoline components, which is directly obtained by crude distillation of light sweet crudes, is insufficient to provide the required volumes of gasoline to the market.
Economic and global political situations dictate many refineries are flexible in processing a wide range of crude oils. Preferred crude oils are those, which are of low cost in the international market. Today the increasing global demand of diesel oils, and the high cost of Brent and other light crude oils forced refineries to shift their distillation strategy from expensive light to cheaper heavy crudes, opportunity crudes and blends thereof.
The introduction of the FCC was a breakthrough in refinery technology. Processing of heavier crudes increases the formation of bottom residues in the atmospheric tower. These undergo further distillation in vacuum to produce LVGO and HVGO. The FCC utilizes the low-cost heavy vacuum distillates to convert them into high-valued gasoline components. This transforma, the revenue of modern FCC units is further expanded by increasing their production capacity of propylene. Many refineries consume their heavy distillates in their own FCC units. In several cases their “own FCC consumption” is too small and excessive heavy distillates are further merchandized at lower price to refineries with insufficient FCC feedstock.
The FCC unit is a complicated complex of different chemical and physical processes, of catalytic cracking, catalyst regeneration and fractionation of the cracked FCC product stream. Inefficient operation of the FCC unit reduces the process yields. This is accompanied by an increase in energy consumption, and an inferior recovery of recycled catalyst.
Production capacity, catalyst utilization and physical properties in FCC process streams are all interlinked, from the vacuum distillates, through the cracked intermediate product until the final distillates from fractionator. Monitoring of physical properties in incoming feed, product streams leaving the reactor, and naphtha and light and heavy oils produced in the fractionators unit. Is the platform for effective overall optimization of the overall FCC process stability, throughput and product quality.
Whereas transparent process streams such as naphtha are controllable by NIR spectroscopy-based technologies. Heavy opaque process streams cannot, which makes NIR spectroscopy being the most suitable technology.
Modern refinery strategy considers to invest in building and expansion of FCC units, to increase their refining margin. The relatively low cost of HVGO and the “own production” in the refineries give the FCC the potential of being a highly profitable unit. To achieve this goal, the FCC must run in its most optimized mode, which is only achieved by stringent control of physical properties in each incoming and outgoing process stream.
Principles of the FCC
During the catalytic cracking process in the FCC, large molecules, which are brought in contact with a fluidized bed catalyst, are cracked into smaller molecules such as C4 – C6, isobutane and others.
Unlike thermal cracking, which induces radical reactions, carbonium ions C+, are involved in the catalytic cracking process. Tertiary carbonium ions are the most stable, while primary carbonium ions are the most labile carbonium ions and easiest to crack. Further reactions that take place during the cracking process in the FCC include isomerization reactions and cracking of longer paraffins in small sized molecules such as C4-C5 molecules. Alkyl benzenes undergo cracking reactions to destroy the paraffinic chains from the aromatic system.
In the FCC reactor, a bed of the alumina – silica catalysts (zeolites) is fluidized by a stream of hot gas, while preheated feedstock is brought in contact with hot catalyst for the reactions to take place. Used catalyst is recycled to the regenerator and recovered by a stream of hot air. Countercurrent steam removes the products from the reactor. The product stream leaving the catalytic reactor is transferred to a distillation tower where gaseous and light naphtha fractions are separated from the heavy distillates, light and cycle oils, LCO and HCO.
The catalytic cracking process produces a broad range of products, which include C3/C4, gasoline, light cycle oil, residual oil and coke. The degree of cracking is traditionally expressed in terms of 430 conversions, defined as 100 minus the volume percentage yields of residual oil and light cycle oil with a boiling point of above 430 F (221°C). Nowadays this definition is arguable as the FCC normally operates at a temperature of between 350 and 550 °C, with a significant yield of product between 220 °C and 350 °C. Therefore, it is preferred to express the yields as percentage of products boiling in the range from C5 – 350 °C. (1)
The cracking yield and the product conversion depend on a number of process variables, such as:
- Reactor temperature
- Feed preheat temperature
- Catalyst circulation rate
- Catalyst Activity
- Recycle Rate
The reactor temperature varies normally between 480 – 550 °C. High temperatures increase the yield of lighter products. Reaction temperatures below 525 °C, produce more gasoline. Raising the temperature to above 525 °C gives with one hand higher-octane gasoline, but on the other hand, higher reactor temperatures cause further cracking which results in an increased amount of light products (overcracking).
Too low preheating reduces the reaction rate and dictates to increase the catalyst-recycling rate. It increases the yields of lights, but also of coke formation. Aside the temperature, other factors that influence the FCC production process can include the residence time of the material in the reaction zone.
Potential FCC feedstock are mainly heavy distillates boiling in the range from 350 – 550 °C similar to HVGO, obtained from the vacuum distillation unit. To exclude contamination by organo-metallic compounds and asphaltenes, direct use of bottom residues from the atmospheric distillation unit and the vacuum distillation process is not recommended.
The cracking pattern for feedstock depends on its composition, which is a mixture of wide range of various aliphatic, aromatic and polycyclic compounds. This makes exact measurement of the composition a difficult task.
From a chemical point of view, aromatics are most difficult to crack. They are generally coke-forming compounds. Naphthens are somewhat more reactive than aromatics. They can undergo ring cleavage to crack easily into olefins, but can also form conversion-inhibiting aromatics. In general, the crack ability of feedstock is inversely proportional to the aromatic carbon atom concentration.
It is also important to know that refinery FCC unit are a source for propylene, which is typically a by-product of gasoline production in larger FCC units. Recently, FCC units are built or modified making propylene production the main objective. Light cycle oil (LCO) is a byproduct of the FCC process that offers the opportunity to produce high-value aromatics. The high level of aromatics, which is present in LCO, makes it suitable for cost-effective conversion to benzene, toluene and xylenes, and other high-value aromatic feedstock.
Product recovery, quality, and product yield.
Vapors leaving the reactor at temperatures of approximately 480 – 550°C are transferred to the fractionator, where they are separated into gas, naphtha, light cycle oil (LCO) and heavy cycle oil (HCO).
The fractionator should provide the requested range of products, with the highest possible volume of valuable gasoline components. To achieve this goal, the reactor must operate under such operating conditions that convert the heavy feed to gasoline components, with the highest chemical yield and capacity. Further to that, the fractionators must operate at such a temperature profile, that provides the best economic cutting points, without affecting the required quality of distillates.
Process optimization of the FCC is an important task to increase refinery revenues and profits by conversion of low valued heavy refinery products into high valued components for gasoline production. It requires operation of the reactor and the fractionator at such process conditions that ensure:
- Minimization of the influence of the quality incoming heavy oil streams, especially upon switching between heavy feeds of different qualities, originated from different refineries, distillates and different crude oils.
- Prevention of contamination by incoming heavy process stream, like metals, sulfur, and heavy residues, also minimizing coke formation.
- Preservation of the catalyst.
- Reduction of the energy consumption, by preventing unnecessary recycling of the catalyst.
- Optimized cracking – maximum naphtha – minimum oils.
- Conformance of the naphtha according to required specification for blending.
FCC process optimization
The FCC is a combination of two different processes, which directly interact between each other.
- Reaction: Cracking of the heavy incoming process stream, including regeneration of the catalyst
- Fractionation: Separates of the cracked product stream into its distillates.
FCC processes contain many variables, which must be controlled to ensure optimized performance and efficiency of the unit. Ongoing and frequent changing of physical properties of process feedstock characterize continuous processes in refineries. Real time analytical data inevitable for immediate adjustment of process parameters following any changes in physical and chemical properties in feed and product process streams. Laboratory analyses are too time consuming to provide effective feedback to the process unit.
Establishment of full process optimization of the reactor and fractionator requires continuous monitoring volumes and qualities of each individual process stream. Real time, and on-line measuring, of their physical and chemical properties is therefore highly important to estimate the degree of conversion of heavy feed into light cracked products.
Process analyzers are divided into:
- Discrete analyzers – Direct measurement of a specified physical property, where each analyzer is dedicated to perform one specific test only.
- Correlative analyzers – Indirect determination of physical properties through chemometric correlation between quantification of spectrometric response signals and quantified physical properties.
The drawback of discrete analyzers is the need of many analyzers to receive a full overview of the process proceedings, which requires an enormous investment in equipment as well as in maintenance.
Correlative analyzers have the advantage that only one single instrument, and one single measurement, is required to quantify instantaneously a broad variety of different physical properties.
Near Infrared (NIR) analyzers are traditionally used correlative analyzers in petroleum industries. They have the feature to perform multi-property remote analyses, as the measuring probe can be allocated far away from the analyzer. Optical fibers transfer a beam of light from the analyzer to the measuring probe. Transmitted light, that passed through the fluid returns to the analyzer. Optical multiplexing enables to measure almost instantaneously a number of different process streams.
Figures 1a,b,c illustrate the accurate correlation between on-line NIR process analytical results for RON, RVP and the T 90% (temperature of distillation 90%) values in FCC gasoline as compared to their corresponding laboratory analyses.
FIGURE 1A: CORRELATION NIR VS LABORATORY MEASUREMENTS OF RON VALUE, IN FCC STREAM
FIGURE 1B: CORRELATION NIR VS LABORATORY MEASUREMENTS OF REID VAPOR PRESSURE (RVP) IN FCC STREAM
FIGURE 1C: CORRELATION NIR VS LABORATORY MEASUREMENTS OF THE TEMPERATURE OF 90% DISTILLATION T90% IN FCC STREAM
Incorporation of NIR with FCC-Process Simulation Models Optimization Software
LP based FCC model simulation software is commercially available on the market. This software calculates the impact of the feedstock and process and operation conditions on parameters such as product yield, catalyst recycling, coke formation. NIR process analyzers have the feasibility to measure physical properties of the feedstock, reactor products and distillation products, which are all directly linked to the production process parameters and operational conditions, and can be utilized to optimize this software.
Chemical compositions and the physicochemical properties in feedstocks are dynamic. Comparison of physicochemical properties of feedstock and product and validation against by the simulation model predicted data is an effective tool to continuously upgrade and improve FCC simulation models. Similar to the integration of process NIR in with LP model simulation software in crude oil blending and CDU optimization(2,3), integration of the NIR with FCC process simulation software enables the complex FCC unit to run in its most profitable and economic mode.
It give the FCC the extra dimension of being even more a moneymaker in refineries than it is already by its initial function to transform low cost distillation vacuum distillates into high valued gasoline. It is superfluous to emphasize that only a short term is required to pay back the investment of the NIR process analyzer (ROI) in the FCC.
Hydrocracking
Within the framework of the application of NIR technologies in FCC, the implementation of NIR process analytics in hydrocracking should also be highlighted. This process is based on hydrogen saturation and cleavage of heavy aromatic aromatic and olefinic molecules. Analogous to the FCC, hydrocrackers crack high molecular weight HVGO predominately into diesel oil, by means of a catalyst and under the influence of pressurized hydrogen. However according to the catalyst product deviation within the range of gas – naphtha – kerosene – distillates are established. Taking into consideration the growing production of heavy vacuum distillates from increased exploration of heavier crudes and opportunity crudes, and the increased demand of diesel oil, the hydrocracker plays definitely an important role in the entire refinery economic structure. Quantification of physical properties of all relevant process streams of the hydrocracker process enables to optimize the process condition by the same concept as employed for FCC optimization. It includes shifting the process towards maximum chemical cracking, highest possible capacity and quality of diesel oil, or other requested cracking compounds, most efficient hydrogen consumption and minimum cost of energy.
Conclusion
In the past, the majority of gasoline was recovered from distillation of light crude oils. The combination of an oil and the high cost of light crudes forced many refineries switching to process heavier crudes. Atmospheric distillation of heavy crudes is accompanied by an increased formation of heavy residues. These are further distilled in vacuum unit into low cost LVGO and HVGO.
Economic and global political situations dictate many refineries to be flexible to process a variety of crude oils, preferably those crude oils that are of low cost in the international market. The development of the FCC process was definitely a breakthrough in refinery economics. It utilizes these low cost vacuum gas oils into gasoline. Many refineries produce more heavy distillates than required for their own consumption in their FCC units. These overproduced distillates are further merchandized at relatively low cost to other refineries, which have enough capacity to process heavy stocks in their FCC, exceeding the quantities produced by their crude distillation units.
Economically, FCC units are financially the paramount of many refineries, and highly beneficial for the entire profit of refineries. To maximize their profitability, refineries are obliged to uninterrupted pursuing the process optimization. Optimized FCC production processes are the result of the economic compromise between maximum chemical yield of naphtha, maximal RON, minimal recycling of the catalyst, minimal coke formation and minimal energy consumption. To achieve this goal, on-line monitoring of the feed, cracked process stream and the distillates is indispensable for efficient adjustment of process conditions. Predictive modeling software for the FCC exist, however chemical processes in the reactor and the fractionators are dynamic, and depend on the fluctuating composition of the feedstock. Integration of NIR analyzers with modeling software enables real time on-line evaluation of chemical and physical properties with predicted parameters. The analytical data is continuously adapted to modify and upgrade the simulation model. This procedure increases the efficiency in the performance of the overall FCC production process and FCC operation. It definitely contributes to an increased catalyst efficiency, and a remarkable reduction in the energy consumption.
Enhanced production, while optimized balancing between the cycle oil production and the gas production is inevitable to guarantee the FCC to operate at lowest cost and at maximum yield and capacity of gasoline components. It is superfluous to indicate that this process will directly increase the profitability not only of the FCC, but also on gasoline production and by that of the entire refinery.